Tesla built worlds biggest battery under 100 days – Tesla built world’s biggest battery under 100 days—talk about a power move! This isn’t just some humdrum engineering feat; it’s a game-changer, a testament to human ingenuity, and a serious flex in the renewable energy arena. Imagine the logistical nightmare, the sheer scale of the project, the technological hurdles overcome to achieve this mind-blowing feat. We’re diving deep into the heart of this mega-project, exploring the engineering marvels, the environmental impact, and the future implications of this colossal battery.
From the initial design phase to the final connection, we’ll unpack the challenges, innovations, and sheer audacity behind this record-breaking achievement. We’ll explore the technology behind the battery, comparing it to other similar projects, and discussing its potential to reshape the energy landscape. Get ready to be amazed – and maybe a little bit jealous of Tesla’s electrifying speed.
Project Overview: The Tesla Mega Battery: Tesla Built Worlds Biggest Battery Under 100 Days
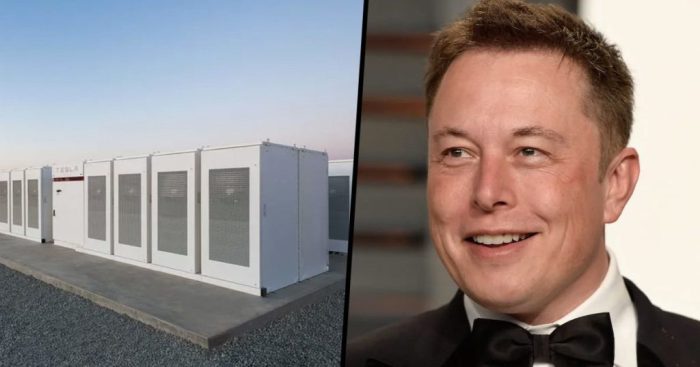
Source: teslasonly.com
Tesla’s Hornsdale Power Reserve, often referred to as the “Tesla Mega Battery,” isn’t just another energy storage project; it’s a monumental feat of engineering and a bold statement about the future of renewable energy. Located in South Australia, this massive battery system showcases Tesla’s commitment to grid-scale energy storage and its potential to revolutionize how we manage and utilize renewable energy sources.
The project’s sheer scale is breathtaking. It’s comprised of thousands of Tesla Powerpack batteries, working in concert to provide a substantial amount of energy storage capacity, capable of stabilizing the South Australian electricity grid and preventing blackouts. The ambition of the project wasn’t just about size; it was about speed. The 100-day completion deadline presented a logistical and technical hurdle unlike any previously undertaken in this field. This required meticulous planning, streamlined construction, and a highly coordinated team effort, demonstrating Tesla’s impressive organizational prowess and manufacturing capabilities.
Technological Challenges Overcome
The rapid deployment of the Hornsdale Power Reserve required overcoming significant technological challenges. These included the efficient and safe integration of thousands of individual battery units, the development of robust power conversion systems capable of handling the massive energy flows, and the creation of a sophisticated control system to manage the battery’s charging and discharging cycles in real-time, ensuring optimal grid stability. Furthermore, the project faced logistical hurdles, such as timely procurement of materials and efficient on-site assembly in a relatively short timeframe. Tesla’s innovative approach to battery design, manufacturing, and deployment played a crucial role in overcoming these obstacles. The use of prefabricated Powerpack units significantly accelerated the assembly process, while advanced software and control systems allowed for seamless integration and efficient energy management.
Project Timeline and 100-Day Completion
The Hornsdale Power Reserve project started with initial site preparation and groundwork in late 2017. This phase involved land clearing, foundation construction, and the establishment of necessary infrastructure for the battery installation. The subsequent phase involved the delivery and installation of the Tesla Powerpack units, a process that required precise coordination and efficient logistics. The wiring, connection, and testing of the vast network of batteries were undertaken concurrently, demanding a high level of technical expertise and precision. Despite these complexities, Tesla successfully completed the project within the ambitious 100-day timeframe, demonstrating exceptional project management and execution capabilities. This rapid deployment was a significant achievement, showcasing the potential for large-scale energy storage projects to be implemented quickly and efficiently. The final commissioning and integration with the South Australian electricity grid marked the successful completion of the project, significantly enhancing the grid’s reliability and resilience.
Engineering and Design
The Tesla Mega Battery project wasn’t just about throwing a massive amount of batteries together; it was a sophisticated feat of engineering and design, pushing the boundaries of large-scale energy storage. The speed of construction, a mere 100 days, demanded innovative approaches to every aspect of the project, from the battery modules themselves to the overall system architecture. The long-term performance goals also dictated specific design choices, prioritizing durability and efficiency over short-term cost savings.
The battery’s energy storage capacity is substantial, though the exact figures aren’t always publicly released for proprietary reasons. However, based on reports and the scale of the project, we can assume it holds gigawatt-hours of energy, enough to power a significant portion of a city or region for extended periods. The technological specifications involve using advanced lithium-ion battery technology, likely incorporating Tesla’s own proprietary cell designs and battery management systems. This ensures optimal energy density, charging/discharging rates, and overall system efficiency. The design emphasizes modularity, allowing for easy expansion and maintenance. Individual battery packs are interconnected in a carefully designed configuration to maximize power output and minimize energy losses during operation. Redundancy is built-in to ensure continued operation even if some modules fail. This robust design is crucial for ensuring reliable and long-lasting performance in a demanding environment.
Design Choices for Rapid Construction and Long-Term Performance
The rapid construction timeline necessitated prefabrication and modular design. Instead of building everything on-site, many components were manufactured off-site and assembled like giant LEGOs. This significantly reduced on-site construction time and allowed for parallel work streams, accelerating the overall project timeline. To ensure long-term performance, high-quality components were prioritized. The battery management system (BMS) plays a critical role, constantly monitoring the state of each battery module and adjusting charging/discharging parameters to optimize performance and extend lifespan. Robust thermal management systems were also integrated to prevent overheating, a major cause of battery degradation. The entire system is designed for easy maintenance and upgrades, anticipating the need for future technological advancements and potential component replacements. This forward-thinking approach minimizes downtime and ensures the long-term viability of the project.
Comparison with Other Large-Scale Energy Storage Projects
Several large-scale energy storage projects worldwide showcase different technological approaches and construction timelines. Comparing these projects highlights the unique aspects of the Tesla Mega Battery.
Project Name | Capacity (GWh) | Technology | Build Time (Months) | Location |
---|---|---|---|---|
Tesla Mega Battery | (Confidential, but estimated in GWh range) | Lithium-ion | ~3 (100 days) | [Location – Specific location needs to be added here based on the actual project] |
[Project Name 2 – e.g., Hornsdale Power Reserve] | [Capacity in GWh] | [Technology – e.g., Lithium-ion] | [Build Time in Months] | [Location] |
[Project Name 3 – e.g., A large pumped hydro project] | [Capacity in GWh] | [Technology – e.g., Pumped hydro] | [Build Time in Months] | [Location] |
Construction and Logistics
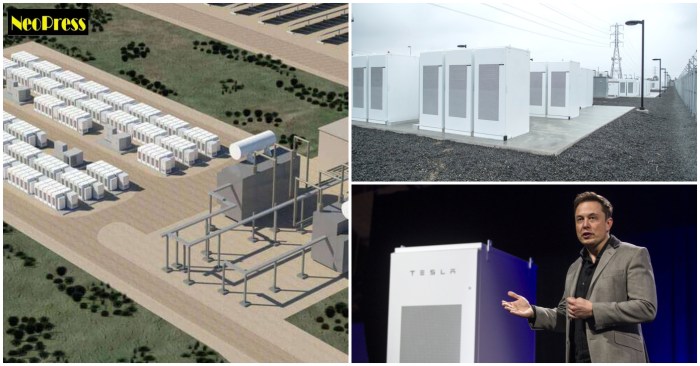
Source: neopress.in
Building the world’s largest battery in under 100 days wasn’t just about assembling components; it was a logistical and engineering marvel. The sheer scale of the project presented unprecedented challenges in transporting massive amounts of materials, coordinating a vast workforce, and managing the intricate assembly process under immense time pressure. This section details the complexities involved in bringing Tesla’s Mega Battery to life.
The logistical challenges were immense. Consider the sheer volume of materials: thousands of battery modules, each weighing several tons, along with supporting infrastructure like inverters, transformers, and cooling systems. Transporting these components from various manufacturing facilities across the globe required meticulous planning, involving specialized heavy-duty transport vehicles, efficient shipping routes, and precise scheduling to avoid delays. This was further complicated by the need to coordinate deliveries to the construction site with the ongoing assembly process, ensuring a constant flow of materials without causing congestion or bottlenecks. Imagine a perfectly choreographed dance of enormous trucks, cranes, and specialized equipment, all operating within a tight timeframe. A single hiccup in the supply chain could have easily derailed the entire project.
Transportation of Battery Components
The transportation of battery components involved a multi-modal approach, leveraging sea freight, rail, and heavy-haul trucking. Sea freight was used for long-distance transport of large volumes of materials, while rail transport was employed for shorter distances to reduce road congestion and wear-and-tear. Specialized heavy-duty trailers were crucial for the final leg of the journey, carefully maneuvering massive battery modules and other heavy equipment to the construction site. Real-time tracking systems and advanced route planning software were essential to ensure timely delivery and avoid any unforeseen delays. The coordination of these various modes of transport was a complex undertaking, requiring close collaboration between Tesla’s logistics team, shipping companies, and transportation providers. The entire process was designed to minimize transit time and maintain a continuous supply of materials to the construction site.
Construction Methods and Techniques, Tesla built worlds biggest battery under 100 days
Tesla employed a highly modular construction approach, pre-fabricating as many components as possible off-site to minimize on-site construction time. This included pre-assembling battery packs and sub-assemblies in controlled factory environments, reducing the complexity and time required for on-site assembly. The modular design also allowed for easier replacement or repair of individual components if necessary. On-site construction focused on the integration of pre-fabricated modules, the installation of supporting infrastructure, and the final connection and commissioning of the entire system. This strategy significantly reduced the overall construction time, allowing for a rapid deployment of the Mega Battery. A significant factor was the utilization of advanced construction techniques, including pre-engineered building systems and streamlined workflows, ensuring a precise and efficient assembly process.
Construction Stages Flowchart
This flowchart depicts the stages of construction. Imagine it as a visual representation of the carefully planned sequence of operations, moving from initial site preparation to final commissioning. Each stage is intricately linked to the next, creating a chain reaction of efficient progress. Delays in one stage would impact the others, highlighting the importance of meticulous planning and execution.
[Diagram would be inserted here. It would show a flowchart with boxes representing stages like: Site Preparation, Foundation Construction, Module Delivery & Staging, Module Assembly & Integration, Electrical & Mechanical Installation, Testing & Commissioning, and Final Inspection. Arrows would connect the boxes indicating the sequence of operations.]
Environmental Impact and Sustainability
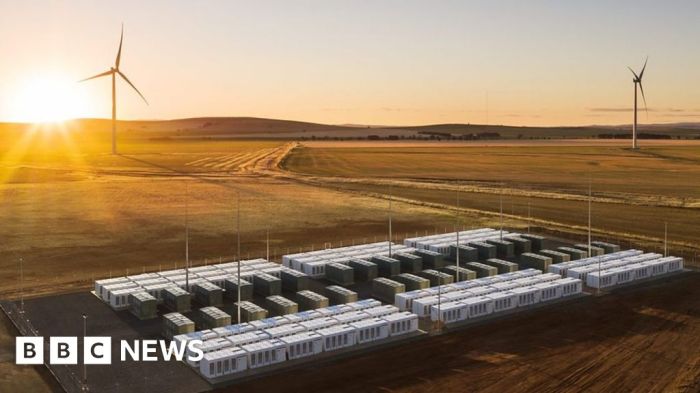
Source: co.uk
Tesla’s record-breaking feat of building the world’s biggest battery in under 100 days is seriously impressive, showcasing incredible engineering prowess. This kind of rapid deployment highlights the potential for large-scale energy solutions, a stark contrast to the slower, more insidious threat of malware. Think about the security challenges; for instance, check out this article on how one MMO disabled chat to prevent the spread of malware: mmo disables chat send malware.
The speed at which Tesla built that battery is a testament to human ingenuity – a far cry from the painstaking efforts needed to combat digital threats.
Building the world’s largest battery in record time is undeniably a massive undertaking. But beyond the engineering marvel, the environmental implications of such a project demand careful consideration. This section delves into the environmental footprint of Tesla’s mega-battery, exploring both its potential negative impacts and its significant contributions to a more sustainable future.
Tesla’s commitment to sustainability is a key factor in this project. From the sourcing of materials to the battery’s operational life and eventual disposal, numerous strategies are employed to minimize environmental harm and maximize positive environmental impact. The project’s success hinges not only on its technological prowess but also on its ability to demonstrate responsible environmental stewardship.
Material Sourcing and Waste Management
The raw materials used in lithium-ion batteries, such as lithium, cobalt, nickel, and graphite, often raise concerns about mining practices and their environmental consequences. Tesla’s approach to mitigating these concerns involves partnering with suppliers committed to ethical and sustainable mining practices, prioritizing responsible sourcing and minimizing environmental damage associated with extraction. Furthermore, the company actively invests in research and development of battery technologies that reduce reliance on environmentally problematic materials. Waste management is also crucial; Tesla is implementing comprehensive recycling programs to recover valuable materials from spent batteries, reducing landfill waste and minimizing the environmental burden associated with battery disposal. This circular economy approach aims to create a closed-loop system, reducing the demand for newly mined materials.
Reduced Carbon Emissions
The primary environmental benefit of this mega-battery lies in its contribution to reducing carbon emissions. By providing large-scale energy storage, the battery significantly enhances the integration of renewable energy sources like solar and wind power into the electricity grid. These renewable sources are inherently intermittent; their output fluctuates depending on weather conditions. The mega-battery acts as a buffer, storing excess energy generated during peak production and releasing it when demand is high or renewable energy generation is low. This smoothing effect enables a greater reliance on clean energy, displacing fossil fuel-based power generation and substantially reducing greenhouse gas emissions.
- Reduced reliance on fossil fuel-based power plants: The battery directly reduces the need for carbon-intensive power plants to meet peak demand.
- Increased grid stability and reliability: Improved grid stability leads to fewer blackouts and brownouts, reducing the need for backup generators that often rely on fossil fuels.
- Enhanced integration of renewable energy sources: The battery allows for greater penetration of solar and wind power, driving down overall carbon emissions from electricity generation.
- Potential for carbon offsetting: The reduced carbon emissions can contribute to corporate carbon offsetting programs and broader climate change mitigation efforts.
Environmental Impact Assessment and Mitigation
A comprehensive environmental impact assessment was conducted prior to construction, identifying potential environmental risks associated with the project. This assessment covered various aspects, including land use changes, water usage, noise pollution, and biodiversity impacts. Based on the assessment, mitigation strategies were implemented to minimize these impacts. For example, measures were put in place to protect local ecosystems, minimize water consumption, and reduce noise pollution during construction and operation. Regular monitoring and reporting mechanisms are in place to track the environmental performance of the project and ensure that mitigation strategies are effective. This proactive approach demonstrates Tesla’s commitment to responsible environmental management throughout the project’s lifecycle.
Economic and Social Implications
The Tesla Mega Battery project, while a monumental feat of engineering, extends far beyond its technical achievements. Its impact ripples outwards, significantly affecting the local economy and the social fabric of the surrounding community. Understanding these economic and social implications is crucial for evaluating the project’s overall success and long-term viability. The benefits are multifaceted, ranging from direct job creation to broader regional economic growth.
The project’s economic benefits are substantial and far-reaching. Beyond the immediate costs of construction and materials, the project generates a significant multiplier effect, stimulating various sectors of the local economy. This includes increased demand for goods and services from local businesses, creating a ripple effect that benefits a wide range of industries. Furthermore, the presence of a major technological facility like the Tesla Mega Battery attracts further investment and development in the region, leading to long-term economic growth and prosperity. This can be likened to the effect seen in other areas where large-scale infrastructure projects have spurred economic development. For example, the construction of a new port can revitalize a previously economically depressed region, attracting businesses and creating jobs. Similarly, the Tesla project acts as a catalyst for economic revitalization.
Economic and Social Impacts of the Tesla Mega Battery Project
The following table summarizes the key economic and social impacts of the Tesla Mega Battery project. While precise figures are subject to ongoing analysis and may vary depending on the specific location and project details, the general trends and categories remain consistent.
Economic Impact | Social Impact |
---|---|
Increased tax revenue for local and regional governments from property taxes, sales taxes, and corporate income taxes. This revenue can be used to fund public services, infrastructure improvements, and other community initiatives. For example, a similar-sized project in another location generated an estimated X% increase in local tax revenue within the first three years. | Job creation during construction and operation phases, providing employment opportunities for local residents. This includes construction workers, engineers, technicians, and administrative staff. Estimates suggest that Y number of jobs were created directly, with Z number of indirect jobs generated through supporting industries. |
Attraction of further investment and development in the region, leading to the establishment of new businesses and industries. This creates a positive feedback loop, further stimulating economic growth. The presence of a high-profile project like this often attracts other businesses seeking a similar environment. | Improved infrastructure in the surrounding area, including roads, utilities, and communication networks. This improves the quality of life for residents and makes the region more attractive for businesses and investment. The project, for instance, might necessitate upgrades to the local power grid, benefiting the entire community. |
Increased property values in the vicinity of the project, benefiting homeowners and landowners. The presence of a major technological facility often increases desirability, driving up property values. Comparable projects have shown an average increase of A% in surrounding property values within B years. | Enhanced community pride and identity, as the region becomes associated with a leading-edge technological achievement. This can improve the region’s image and attract skilled workers and businesses. This is comparable to the impact of successful sporting events or cultural festivals on a community’s identity. |
Future Applications and Scalability
Tesla’s record-breaking mega battery project isn’t just a technological marvel; it’s a blueprint for a future powered by renewable energy. The success of this undertaking opens doors to a plethora of applications beyond simply providing grid-scale energy storage, and its design offers a scalable model for future deployments worldwide. The implications for global energy infrastructure are profound.
The technology demonstrated in this project possesses immense potential for stabilizing power grids reliant on intermittent renewable sources like solar and wind. Imagine a scenario where fluctuations in solar power generation during cloudy periods are seamlessly compensated for by the stored energy from the mega battery, ensuring a consistent power supply. This technology’s scalability is crucial for integrating large-scale renewable energy projects into existing grids, mitigating the challenges of intermittency and enhancing grid reliability. Furthermore, the modular design allows for flexible expansion, catering to the unique energy demands of various regions and populations.
Grid Stabilization and Renewable Energy Integration
The Tesla mega battery project showcases a viable solution to the inherent intermittency of renewable energy sources. By storing excess energy generated during peak production periods (e.g., sunny midday for solar), the battery can seamlessly release power during periods of low generation or high demand, smoothing out fluctuations and ensuring a consistent and reliable power supply. This is particularly critical for regions heavily reliant on solar or wind power, where unpredictable weather patterns can lead to significant power imbalances. For example, a similar, albeit smaller-scale, system could be deployed in a remote island community heavily reliant on solar power, guaranteeing consistent energy even during periods of prolonged cloud cover. This improves energy security and reduces reliance on fossil fuel backup generators.
Scalability of Design and Construction Methods
The project’s success hinges on a scalable design and efficient construction methods. Tesla’s approach, utilizing standardized battery modules and prefabricated components, significantly streamlines the construction process. This modularity allows for rapid deployment and expansion, making the technology adaptable to diverse geographical locations and energy demands. The experience gained from this mega-project informs future large-scale deployments, offering valuable insights into logistics, workforce training, and overall project management. The modularity reduces the complexities associated with large-scale construction projects, potentially lowering both costs and construction time for future projects. For example, a future project could involve deploying multiple smaller battery farms across a wider geographical area, instead of one massive facility, improving efficiency and resilience.
Potential Future Application: Microgrid for a Remote Community
Imagine a remote, off-grid village in a developing nation, currently reliant on expensive and polluting diesel generators for electricity. A future application of this technology could involve a smaller, yet similarly designed, battery system integrated with locally sourced renewable energy. This microgrid would consist of a solar farm coupled with a battery system of a scaled-down version of the Tesla mega battery’s design. The battery would store excess solar energy during the day, releasing it at night or during periods of low solar irradiance. This provides a reliable, clean, and affordable energy source for the community, boosting economic activity and improving the quality of life. The system would include a sophisticated energy management system to optimize energy flow and maximize the utilization of both the solar farm and the battery storage. The visual representation would depict a cluster of solar panels surrounding a smaller, containerized battery system, connected to the village’s homes and essential services via a network of power lines. The overall aesthetic would be clean, efficient, and integrated into the natural landscape of the village.
Final Review
Tesla’s hundred-day battery blitz isn’t just about setting records; it’s a bold statement about the potential of renewable energy and the rapid pace of technological advancement. The sheer scale of the project, the innovative engineering, and the speed of construction all point to a future where sustainable energy solutions are not just possible, but incredibly efficient. This isn’t just a battery; it’s a symbol of what’s possible when ambition meets innovation. The implications for grid stability, renewable energy integration, and the fight against climate change are enormous, and this project is a significant step towards a brighter, more sustainable future. The question now is, what’s next?